
貝克曼庫爾特商貿(mào)(中國)有限公司
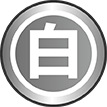
已認證
摘要
小球藻因其高蛋白質(zhì)含量和與世界衛(wèi)生組織(WHO)推薦的人類營養(yǎng)素相似的氨基酸譜,以及富含人體必需營養(yǎng)素,廣泛應用于食品和飼料工業(yè)領域1。此外,小球藻在不利的生長條件下可積累C16和C18脂肪酸2,3,為污水處理廠生產(chǎn)生物燃料提供了可能1。
我們將為您展示搭載LAM的BioLector XT高通量微型生物反應器,適用于小球藻的光自養(yǎng)培養(yǎng)。此外,專門設計并驗證的濾光片模塊支持多種關鍵培養(yǎng)參數(shù)的在線監(jiān)測。
概述
通常,小球藻是通過搖瓶在有限的實驗通量下進行培養(yǎng)。培養(yǎng)條件優(yōu)化、藻株篩選和藻株工程是商業(yè)化的三大關鍵步驟4,例如,通過縮短葉綠素天線尺寸以最大限度地提高太陽能-產(chǎn)物能量轉換效率5。基于微孔的培養(yǎng)設備為微生物和哺乳動物細胞早期快速篩選提供了一種強大的工具6,并已成功應用于異養(yǎng)微藻生物工藝開發(fā)7。
微型光照生物反應器(μPBR)系統(tǒng),可用于研究自養(yǎng)或混合營養(yǎng)培養(yǎng)條件,但目前的概念離系列技術還很遠,進一步的改進——尤其是可調(diào)節(jié)照明——是必要的8,9。
搭載精密光照模塊的BioLector XT高通量微型生物反應器,可在多達48個微孔中同時進行光營養(yǎng)培養(yǎng)。通過專門設計的濾光片模塊,可實現(xiàn)非侵入式、實時在線監(jiān)測關鍵培養(yǎng)參數(shù)如生物量、葉綠素濃度和pH值。光照模塊(LAM)在光合光譜范圍內(nèi)提供精確、多樣化的光照方案。其光譜的靈活性是通過16種不同的LED實現(xiàn)的,每一個LED都可以單獨控制,可提供近4000 μmol/m2/s的最大輻射。
方法
濾光片模塊開發(fā)
經(jīng)專門設計并測試的濾光片模塊,可用于在線監(jiān)測多種重要的光營養(yǎng)培養(yǎng)參數(shù)(生物量、葉綠素含量和pH值)。
小球藻
無菌小球藻(SAG藻株編號211-11b)由哥廷根大學藻種保藏中心提供。
培養(yǎng)基
在pH值為6.5的改良Bold基礎培養(yǎng)基(enBBM)中進行培養(yǎng)10-13。
在搖瓶中預培養(yǎng)
將小球藻置入振幅為50mm的搖瓶中,在25℃培養(yǎng)箱中以180rpm振速進行預培養(yǎng)。為實現(xiàn)光營養(yǎng)生長,在培養(yǎng)室的一側安裝LED模塊,同時在搖瓶口使用棉塞,方便氣體出入培養(yǎng)液。LED模塊由8個平行安裝的模擬太陽光LED燈條組成(LUMITRONIX LED-Technik GmbH)。將輻照度設置為200μmol/m2/s,使之與BioLector XT微型生物反應器培養(yǎng)時的輻照度一致。
在BioLector XT微型生物反應器中培養(yǎng)
采用Flowerplate梅花板[M2P-MTP-48-B]進行光營養(yǎng)培養(yǎng),初始細胞密度為5.5*106個細胞/mL,培養(yǎng)體積為1mL。用透氣性密封膜(MTP-F-GPRS-48-10)密封微孔板,方便光合作用期間二氧化碳和氧氣的交換。照明模塊設置為提供400~700nm的類太陽光譜,光子通量密度約為200 μmol/m2/s(圖1)。
圖1. 培養(yǎng)期間的照明光譜和輻照度
將培養(yǎng)參數(shù)設置為800 rpm、25℃和85% 的相對濕度,并按10 mL/min流速充入空氣和 2% 的CO2混合物。
結果
濾光片模塊校準
圖2.濾光片模塊校準結果圖組
生物量
經(jīng)專門開發(fā)的730、750和850 nm生物量濾光片模塊,可在0.3≦OD750≦25范圍內(nèi)顯示出卓越的校準效果,730 nm模塊校準結果如圖2.A所示。此外,使用葉綠素a和葉綠素b標準品,未發(fā)現(xiàn)葉綠素干擾。
葉綠素含量
葉綠素校準結果顯示,經(jīng)專門設計的濾光片模塊可單獨或同時檢測葉綠素a和葉綠素b,如圖2.B所示。
葉綠素熒光常用于測定光營養(yǎng)培養(yǎng)物的生物量14,15。因此,使用生物量系列稀釋液進行校準,應用指數(shù)遞減擬合時,在低生物量濃度下可觀察到極佳的分辨率如圖2.C所示。校準結果證實,葉綠素熒光濾光片器可準確測定生物量濃度,特別是在生物量濃度較低的時候。
pH值
分別在25、30、35和40℃下,使用含0.1 mg/L HPTS的pH 5~10緩沖溶液(Merck, Darmstadt, DE)對pH濾光片模塊進行校準。校準結果出色(圖2.D),證明所該設置適用于在線準確測定pH值。
為確保擬定方法對光漂白不敏感,我們在BioLector XT微型生物反應器中使用無接種物enBBM在200μmol/m2/s典型培養(yǎng)條件進行培養(yǎng)實驗(圖2.E)。實驗開始后,CO2濃度的增加使培養(yǎng)基迅速酸化(~0.05pH),但在之后的130小時內(nèi),測量值(圖2.E)始終保持恒定。這一點證明,在連續(xù)光照的培養(yǎng)條件下,使用HPTS測定pH,可連續(xù)多日保持準確、穩(wěn)定的測量水平。
在BioLector XT微型生物反應器中并行光營養(yǎng)培養(yǎng)
經(jīng)過對濾光片模塊進行驗證之后,我們開始長期培養(yǎng),并使用四個濾光片模塊進行在線監(jiān)測:730nm的散射光模塊,葉綠素熒光濾光片模塊(λex=450nm;λem=700nm)和兩種HPTS濾光片模塊進行比值pH測定(圖3)
圖3. 搭載光照模塊的BioLector XT微型生物反應器,為期16天的培養(yǎng)中所監(jiān)測的散射光、葉綠素和pH值
如圖3所示,為期16天的培養(yǎng)監(jiān)測中,生物量濃度持續(xù)增加,直至CO2供應被關閉。圖中可觀察到五個不同的生長階段:
首先是滯后的指數(shù)生長期(i.),之后是三個不同的線性生長期(II.至IV.),最后是CO2耗盡后進入的死亡階段(V.)。pH和葉綠素信號的變化過程與觀察到的生長階段相關。在整個實驗過程中,散射光信號的平均變異系數(shù)為5.2%,因此可在建議的設置中進行平行光營養(yǎng)培養(yǎng)。離線樣本驗證了在線信號的準確性。235小時后采樣(此時由于短暫暴露于大氣中的CO2濃度中,pH值升高),離線OD750值為40,離線pH值為7.3,這與在線測定的pH值7.2高度一致。在實驗結束時,離線OD750值為60左右,表明在實驗過程中培養(yǎng)物生長旺盛導致產(chǎn)生較高的生物量濃度。
結論
光照模塊的加入為BioLector開創(chuàng)了全新的應用領域。本文應用案例中所介紹的幾種濾光片模塊,可準確監(jiān)測關鍵的光營養(yǎng)培養(yǎng)參數(shù),如生物量濃度、葉綠素含量和pH值。
葉綠素熒光濾光片模塊在低濃度生物量測定中具有良好的分辨率,與在高細胞密度下準確的散射光測量相得益彰16。此外,研究證明,基于HPTS的pH測定法是替代optode測量的理想解決方案,即便在連續(xù)照明的條件下,該方法也同樣適用。
小球藻的長期培養(yǎng)證實,BioLector系統(tǒng)可用作并行、高通量培養(yǎng)的微升級光照生物反應器。此外,經(jīng)專門設計的濾光片模塊可評估培養(yǎng)物生長的在線信息,并揭示不同的生長階段。
這些結果證實該平臺可通過不同方式優(yōu)化各種應用的光營養(yǎng)培養(yǎng)。精心設計的光照模塊,支持在不同照明條件下設置較寬的光譜和輻照度范圍,以便優(yōu)化照明條件。多篇文獻已報道這些照明特性可影響藻類生長9,17-21。
在BioLector XT中,可通過氣體流量和氣體成分改變實驗的氣體培養(yǎng)條件,這是光營養(yǎng)培養(yǎng)中的一個重要參數(shù),例如,CO2富集可獲得更高的生物量濃度1,19。其他影響微藻培養(yǎng)的關鍵因素包括溫度22,23、pH1,20、鹽度24、碳源25-27、氮源19,27和培養(yǎng)基成分28。BioLector XT可優(yōu)化所有這些參數(shù)。此外,建議采用分批補料培養(yǎng)來提高脂質(zhì)產(chǎn)量29。由于監(jiān)管限制,有關基因工程藻株潛力的研究和應用極少,但藻株經(jīng)基因改造,可能具有更高的生長速率和細胞密度,更高的生產(chǎn)速率或滴度,更強的魯棒性或更好的太陽能-生物量轉換效率和光合生產(chǎn)力30,5,31,32。
所有這些因素均可通過搭載LAM的BioLector XT微型生物反應器進行研究和優(yōu)化,助力研究人員加快研究進程,為實現(xiàn)藻類潛能所需的答案提供幫助。
參考文獻:
1. Ru, I.T.K., et al., Chlorella vulgaris: a perspective on its potential for combining high biomass with high value bioproducts.Applied Phycology, 2020. 1(1): p. 2-11.2. Yeh, K.-L. and J.-S. Chang, Effects of cultivation conditions and media composition on cell growth and lipid productivity ofindigenous microalga Chlorella vulgaris ESP-31. Bioresource technology, 2012. 105: p. 120-127.3. Maruyama, I., et al., Application of unicellular algae Chlorella vulgaris for the mass-culture of marine rotifer Brachionus, inLive Food in Aquaculture. 1997, Springer. p. 133-138.4. Chisti, Y., Constraints to commercialization of algal fuels. Journal of biotechnology, 2013. 167(3): p. 201-214.5. Melis, A., Solar energy conversion efficiencies in photosynthesis: minimizing the chlorophyll antennae to maximizeefficiency. Plant science, 2009. 177(4): p. 272-280.6. Ojo, E.O., et al., Design and parallelisation of a miniature photobioreactor platform for microalgal culture evaluation andoptimisation. Biochemical Engineering Journal, 2015. 103: p. 93-102.7. Hillig, F., et al., Bioprocess Development in Single-Use Systems for Heterotrophic Marine Microalgae. Chemie IngenieurTechnik, 2013. 85(1-2): p. 153-161.8. Morschett, H., et al., Laboratory-scale photobiotechnology-current trends and future perspectives. FEMS Microbiol Lett,2018. 365(1).9. Kiss, B. and á. Németh, High-throughput microalgae cultivation with adjustable LED-module applying different colours forNannochloropsis and Chlorella microcultures. Acta Alimentaria, 2019. 48(1): p. 115-124.10. Bischoff, H.W. and H.C. Bold, Some soil algae from Enchanted Rock and related algal species. 1963, Austin, Tex.: Universityof Texas.11. Bold, H.C., The Morphology of Chlamydomonas chlamydogama, Sp. Nov. Bulletin of the Torrey Botanical Club, 1949. 76(2):p. 101-108.12. Andersen, R.A., Algal culturing techniques. 2005, Burlington, Mass.: Elsevier/Academic Press.13. Morschett, H., W. Wiechert, and M. Oldiges, Accelerated Development of Phototrophic Bioprocesses: A ConceptualFramework. 2017, RWTH Aachen University.14. Wiltshire, K.H., et al., The determination of algal biomass (as chlorophyll) in suspended matter from the Elbe estuary andthe German Bight: A comparison of high-performance liquid chromatography, delayed fluorescence and promptfluorescence methods. Journal of Experimental Marine Biology and Ecology, 1998. 222(1): p. 113-131.15. Ramaraj, R., D.D. Tsai, and P.H. Chen, Chlorophyll is not accurate measurement for algal biomass. Chiang Mai Journal ofScience, 2013. 40(4): p. 547-555.16. m2p-labs GmbH, Baesweiler Germany, The scattered light signal: Calibration of biomass. 2015.17. Carvalho, A.P., et al., Light requirements in microalgal photobioreactors: an overview of biophotonic aspects. AppliedMicrobiology and Biotechnology, 2011. 89(5): p. 1275-1288.18. Johnson, T.J., et al., Photobioreactor cultivation strategies for microalgae and cyanobacteria. Biotechnology Progress,2018. 34(4): p. 811-827.19. Daliry, S., et al., Investigation of optimal condition for Chlorella vulgaris microalgae growth. Global journal of environmentalscience and management, 2017. 3(2).20. Gong, Q., et al., Effects of Light and pH on Cell Density of Chlorella Vulgaris. Energy Procedia, 2014. 61: p. 2012-2015.21. Sforza, E., et al., Adjusted Light and Dark Cycles Can Optimize Photosynthetic Efficiency in Algae Growing inPhotobioreactors. PLoS ONE, 2012. 7(6): p. e38975.22. Serra-Maia, R., et al., Influence of temperature on Chlorella vulgaris growth and mortality rates in a photobioreactor. AlgalResearch, 2016. 18: p. 352-359.23. Converti, A., et al., Effect of temperature and nitrogen concentration on the growth and lipid content of Nannochloropsisoculata and Chlorella vulgaris for biodiesel production. Chemical Engineering and Processing: Process Intensification,2009. 48(6): p. 1146-1151.24. Minhas, A.K., et al., A Review on the Assessment of Stress Conditions for Simultaneous Production of Microalgal Lipids andCarotenoids. Frontiers in Microbiology, 2016. 7.25. Kong, W., et al., The characteristics of biomass production, lipid accumulation and chlorophyll biosynthesis of Chlorellavulgaris under mixotrophic cultivation. African Journal of Biotechnology, 2011. 10(55): p. 11620-11630.26. Scarsella, M., et al., Study on the optimal growing conditions of Chlorella vulgaris in bubble column photobioreactors.Chem. Eng, 2010. 20: p. 85-90.27. Heredia-Arroyo, T., et al., Mixotrophic cultivation of Chlorella vulgaris and its potential application for the oil accumulationfrom non-sugar materials. Biomass and Bioenergy, 2011. 35(5): p. 2245-2253.28. Blair, M.F., B. Kokabian, and V.G. Gude, Light and growth medium effect on Chlorella vulgaris biomass production. Journalof Environmental Chemical Engineering, 2014. 2(1): p. 665-674.29. Keil, T., et al., Polymer-based ammonium-limited fed-batch cultivation in shake flasks improves lipid productivity of themicroalga Chlorella vulgaris. Bioresour Technol, 2019. 291: p. 121821.30. Hallmann, A., Algae biotechnology–green cell-factories on the rise. Current Biotechnology, 2015. 4(4): p. 389-415.31. Fayyaz, M., et al., Genetic engineering of microalgae for enhanced biorefinery capabilities. Biotechnology Advances, 2020.43: p. 107554.32. Ng, I.S., et al., Recent Developments on Genetic Engineering of Microalgae for Biofuels and Bio-Based Chemicals.Biotechnology Journal, 2017. 12(10): p. 1600644.
最新動態(tài)
更多